https://x.com/_HannahRitchie/status/1744616558125609179
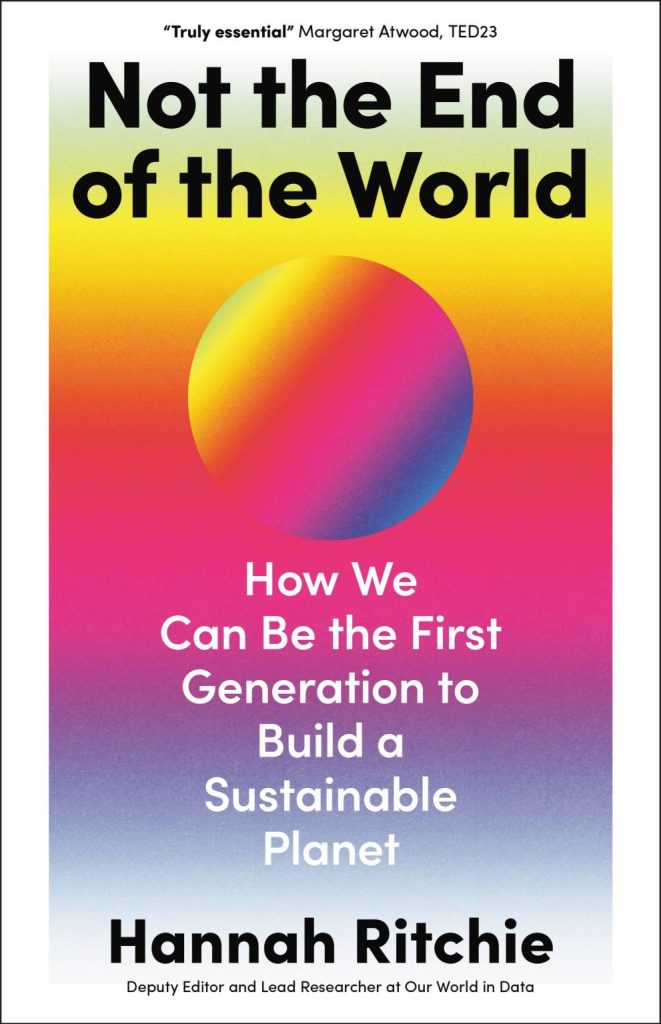
Recommend this one
We have a new paper out in The Journal of Power Sources, which is a collaboration with Energizer. We use high energy white beam tomography to study the distribution of ZnO in the anodes of cylindrical alkaline batteries. (These are AAA, but we also studied AA sizes.) The finding is that the distribution of ZnO is a strong function of how the battery was discharged. In the image below, batteries (d) and (e) were discharged to the same depth (295 mAh) at the same rate (21 mA).
Continuous discharge (e) matched what you expect from a computational battery model: relatively dense ZnO (pink) found mostly near the separator, around the anode’s circumference. However, if the discharge was pulsed (d), the ZnO had a very low density (blue) and was mostly in large clumps in the anode interior. Since most primary batteries are used in an intermittent or pulsed manner, understanding how this affects where the resistive ZnO is located is important for getting more capacity out of the cells. We found that varying the ZnO density helps reconcile computational models with experimental results.
PhD student Dom Guida came up with a custom segmentation method to analyze these data. All the details are in the paper and the supplemental info. This tomography was special because the resolution was good (a little less than 3 microns) with a quite large field of view, letting us use unaltered AA and AAA cells and record the entire battery diameter.
“Lithiation Gradients and Tortuosity Factors in Thick NMC111-Argyrodite Solid-State Cathodes” Our new work in ACS Energy Letters. Spatial Li gradients change a lot in all-solid-state batteries with NMC loading.
By matching operando synchrotron diffraction data to a computational battery model, we back out the electrode tortuosity factor. In these cells it ranges from 7-25 (much larger than the 2-4 in typical Li-ion cell with liquid electrolyte).
We have a new paper in The Journal of Physical Chemistry C, written by Dr. Bebi Patil. It deals with composite solid electrolytes (CSEs) based on (1) a Li-conducting filler (LATP) in (2) a polymer matrix (PEO + LiTFSI). This work demonstrates that engineering fillers to have space-filling shapes has a profound positive impact on conductivity of the resulting CSE. Our approach was to engineer a micron-scale filler with a hollow-sphere morphology (shown below) because hollow spheres fill space efficiently and shift agglomeration to the level of the secondary particles. Also the hollow spheres are porous and have both an inner and outer surface.
With CSEs, it is of paramount importance for the ceramic filler to present maximum surface area to the polymer matrix. This is typically done by exploiting the high surface area to volume ratio of nanomaterials. To achieve a percolated Li-conducting interphase across the electrolyte, many groups have engineered fillers in a nanofiber or nanorod morphology. However, our micron-scale hollow sphere material performed as well as or better than nanoscale materials. It had a near-record room temperature conductivity (1.64 × 10-4 S/cm) for a PEO/LATP-based CSE. (This is a good start, but conductivity still needs to be higher for CSEs to be widely used in battery products.)
Li metal batteries based on CSEs are desired because polymer processing would be simpler to integrate into battery manufacturing than the high temperature methods required for ceramic solid electrolytes. Also, polymers make good interfaces with battery active materials. The challenge is that their Li conductivities are generally much lower than other solid electrolytes (like sulfides or garnets). Including a filler material improves the conductivity. There are several reasons for this, some of which are not completely understood.
Northeastern student Marisol Chang Zapata designed us a wonderful cover image!